The Uncertainty of Detecting Planets
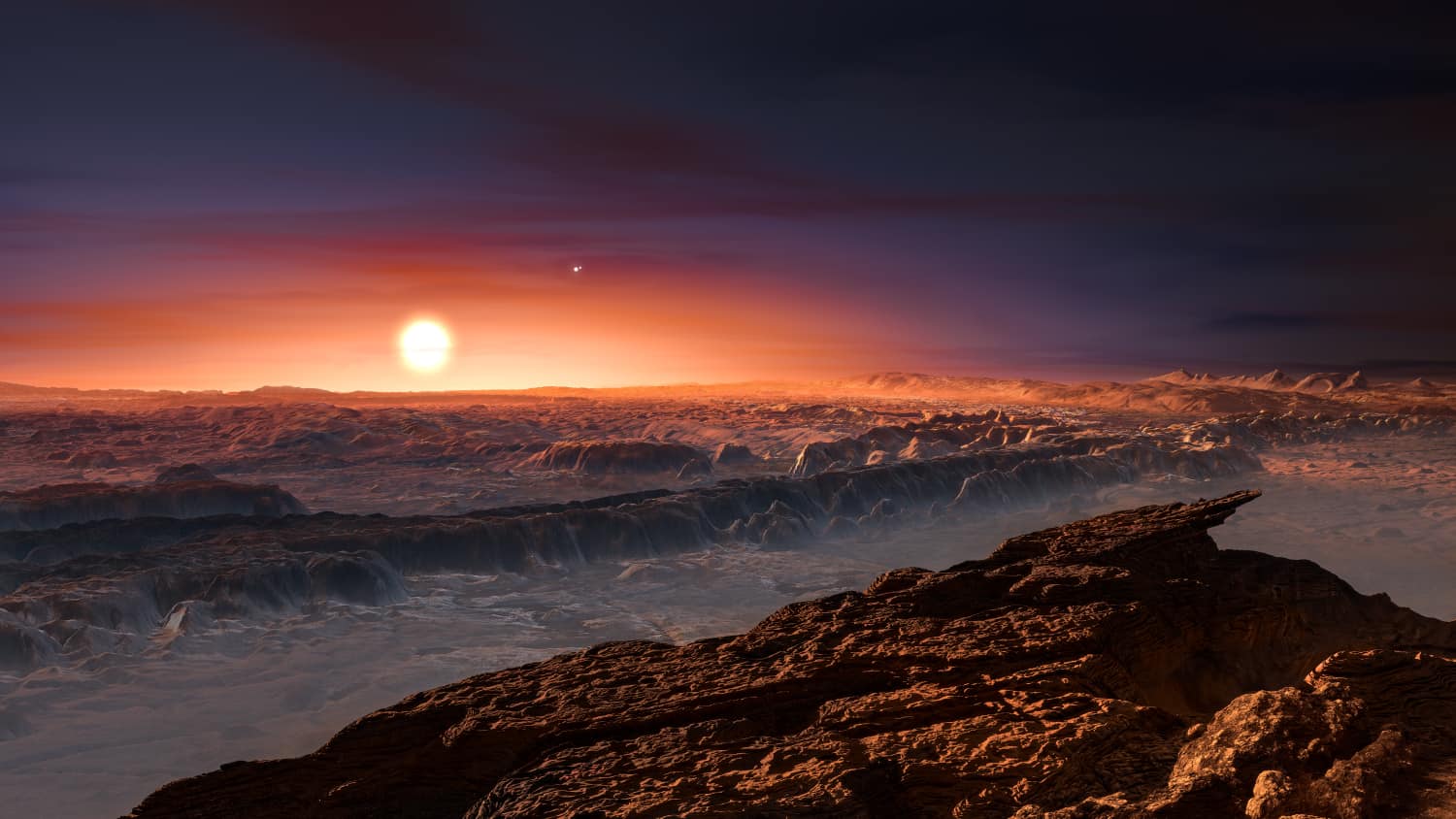
Editor’s Note: This is a guest post by Paul Byrne, a planetary geologist and assistant professor in NC State’s Department of Marine, Earth, and Atmospheric Sciences. This post is part of a series that addresses uncertainty in science. Uncertainty is a topic that is often misunderstood by people outside of the research community, and people often think that uncertainty means researchers have no idea what is true. This series aims to address these misconceptions about uncertainty in research.
Uncertainty in science is a good thing. Because here’s how the scientific model works: you observe a phenomenon, then form a hypothesis about why that phenomenon is taking place, then test the hypothesis, which leads you to develop a new hypothesis, and so on. That process means it can be difficult to ever definitely know something. Instead, scientists work to understand the uncertainty in their measurements, their models, their conclusions.
In other words, rather than being a limitation, uncertainty can help improve our knowledge of the natural world, and tell us what questions to ask next.
But that comfort with uncertainty doesn’t always translate to how scientific findings are communicated. Especially with pervasive social media and fast turnaround times for journalists and press offices, the nuances or even the major limitations of a scientific discovery can be hard to convey to the public. As a result, it’s possible for people to – entirely understandably – get the impression that a new finding is more robust than it really is.
Take exoplanets. Once thought to be confined to the realm of science fiction, there are now more than 4,000 worlds known to orbit other stars. And that number is constantly rising. Most excitingly, spacecraft like NASA’s TESS mission are increasingly capable of searching for smaller, rocky exoplanets, including those that might be Earth-like and perhaps even habitable.
There are several methods by which exoplanets are detected. Suspected exoplanets are called “candidates” until two or preferably more independent approaches confirm that they are, in fact, real. The two primary techniques are transit photometry and the radial velocity method.
Transit photometry involves observing a distant star through a telescope (usually a very powerful one) and watching to see if its brightness dims. If so, one explanation for that dimming is that a planet passed between the star and the observer on Earth. If a star seems to dim regularly, that’s good circumstantial evidence that a planet crossing in front of the star is the culprit. Transit photometry can even estimate the size of a planet, by measuring how much the planet dims its star (because a bigger planet will block more light than a smaller planet).
Of course, for this method to work, the orbital plane of an exoplanet must be such that it crosses the star as viewed by Earth. And the planet must circle its star frequently enough that we can detect it in a reasonable amount of observing time. For example, a planet that takes as long to go around a star as Pluto takes to orbit the Sun isn’t something we’re likely to detect, even if its orbital plane is edge-on to Earth.
The radial velocity method looks for tiny wobbles in a star’s rotation (as measured by variations in the properties of the light it emits). As is the case for transit photometry, if this wobble occurs regularly, then we might reasonably conclude that the gravitational tug of an orbiting planet is responsible. And, again, this wobble needs to repeat frequently enough that we stand a chance of picking it up with telescopes.
However, a major advantage of the radial velocity method over transit photometry is that a planet need not cross its star from the perspective of an astronomer on Earth. But that’s also where a major uncertainty lies in understanding the kind of planet we might detect with this method.
Imagine a planet circling its star in an orbit that’s edge on, relative to Earth. The wobble this planet would induce in its star would be a maximum value from our perspective: the amount the star would move is greatest toward or away from us. (Of course, the amount of this motion is truly tiny, but something we can still measure with modern telescopes.). On the other hand, if the planet orbited in a plane that was face-on to us – that is, we would see the entire orbit as a circle from our vantage point – then we wouldn’t see any wobble at all. All the tugging on the star would be in the plane of the orbit, leaving no change in the properties of the light of the star for us to detect.
But what if, as is most likely, a planet orbits in a plane that is neither edge on, nor face on, to us?
The wobble we’d detect would be a portion of the total wobble. And since the magnitude of the wobble relates to the mass of the orbiting planet, we would only be able to measure a minimum value for the mass of that planet. This matters, because mass equates to size: a low-mass planet has a higher chance of being rocky than a high-mass planet. And here’s where multiple detection methods are helpful, because if transit photometry can measure a planet’s size, and radial velocity measurements give us the planet’s mass, then the density of the exoplanet can be calculated.
A planet with a high density is much more likely to be rocky – like Earth or Venus – than a planet with a lower density, which could be composed mainly of gases, such as Neptune and Uranus. But for an exoplanet detected with radial velocity alone, it can be impossible to know if its measured mass value is accurate, and so the nature of such a planet, rock or gaseous, is uncertain.
Astronomers know this, of course, and unless the angle of a planet’s orbit relative to Earth is known (with transit photometry, say), they report the mass of an exoplanet found with the radial velocity method as a minimum. This is an example of where uncertainty in science is fully acknowledged. But it’s also an example of where that uncertainty isn’t necessarily obvious to someone not particularly familiar with how exoplanets are discovered.
For example, in 2016, the European Southern Observatory announced the discovery of a planet orbiting the nearest star to the Sun, Proxima Centauri. This planet, named Proxima b, was detected with the radial velocity method and has a minimum mass of 1.27 times that of Earth, making it a rocky planet. (You can see an artist’s impression of the planet at the top of this page.)
But it’s entirely possible that Proxima b is more massive still, and could even be a mini-Neptune – a type of planet not found in our solar system, but that seems to be common elsewhere, with a thick hydrogen–helium atmosphere. A mini-Neptune looks nothing like a rocky world such as Earth, but the illustrations that accompanied news of Proxima b’s discovery (like the one at the top of this page) couldn’t easily capture that uncertainty. And so, although exoplanets are incredibly exciting things to study and learn about, it’s worth keeping an open mind when articles crop up about the potential habitability of planets that are just next door to us. At least, until we can actually pay them a visit.
This post was originally published in NC State News.